Higher-level only
The first row of the 'd' block elements excluding scandium and zinc are known as transition metals. They share common characteristics that arise from having similar atomic and ionic radii.
Syllabus ref: S3.1.8Structure 3.1.8 - Transition elements have incomplete d-sublevels that give them characteristic properties. (HL)
- Recognize properties, including: variable oxidation state, high melting points, magnetic properties, catalytic properties, formation of coloured compounds and formation of complex ions with ligands
Guidance
- Knowledge of different types of magnetism will not be assessed.
Tools and links
- Nature of science, Structure 2.3 - What are the arguments for and against including scandium as a transition element?
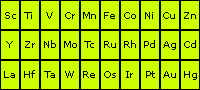
Definition of transition metals
The term transition originally meant the elements that formed the transition between the 's' block and the 'p' block of the periodic table. However, it has since come to mean the elements which have compounds containing partiallly filled 'd' orbitals.
By this definition, in the first row of the transition metals the elements scandium and zinc are not included:
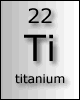
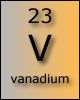
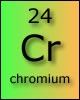
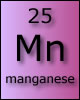
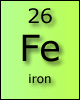
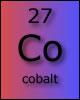
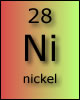
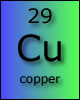
Scandium, the element, has an electronic configuration [Ar] 4s2 3d1, but it only forms ions with a 3+ charge, with a configuration of [Ar] 4s0 3d0.
Zinc, on the other hand, has a configuration with a full set of 'd' orbitals [Ar] 4s2 3d10, and only forms 2+ ions, in which the electronic arrangement is [Ar]4s0 3d10. Hence, scandium and zinc do not display many of the properties characteristic of the transition metals and are not included.
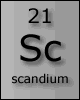
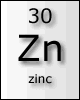
Physical properties
The '3d' sub-shell is inside the 4s sub-shell, meaning that as it gets filled moving from element to element, the inter-electron repulsion shields the outer 4s electrons from the increased nuclear charge. The consequence is that the atomic radius does not change as much as when crossing a short period, such as period 2 or 3.
Atomic radius initially shows a decrease from titanium to chromium, but once the electrons start to pack into the inner '3d' sub-shell the radius remains fairly constant.
The transition metals share common physical properties due to the contribution of their 3d electrons to delocalisation and metallic bonding.
- Hard
- High melting point
- High electrical conductivity
- High thermal conductivity
The elements are hard metals with high melting points, a characteristic of strong metallic bonding.
Electronic configurations
The 4s orbitals fill up before the 3d orbitals, according to Hund's rule and the Aufbau principle, but there are two exceptions in the cases of chromium and copper.
Chromium half-fills its 'd' orbitals by promoting an electron from the 4s orbital. This gives rise to a half-filled 'd' shell that has some inherent stability, or at least enough extra stability to compensate for the energy needed to promote the 4s electron.
Copper similarly promotes one 4s electron to achieve a full set of 'd' orbitals.
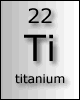
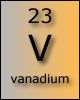
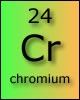
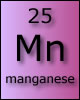
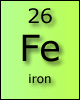
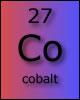
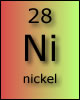
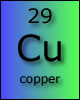
Ion formation
Transition elements form positive ions by loss of electrons. The first electrons lost are the 4s electrons. Although this may seem counter intuitive as the last electrons to enter were the 3d, it seems as though the act of filling the '3d' sub-shell lowers its energy allowing the '4s' electrons to be lost first.
Example: Show the electronic configurations of an Fe atom and the Fe2+ ion.
- Fe atom - [Ar] 4s2 3d6
- Fe2+ ion - [Ar] 4s0 3d6
The common oxidation state of the transition elements is +2, reflecting the loss of the two electrons from the 4s orbital. Chromium's most stable oxidation state is not +2, however, reflecting the fact that there is only one 4s electron.
Once the 4s electrons have been lost, further loss of 3d electrons can lead to higher oxidation states.
Example: Show the electronic configurations of vanadium and the vanadium +2, +3, +4 and +5 oxidation states.
- V atom - [Ar] 4s2 3d3
- V(II) - [Ar] 4s0 3d3
- V(III) - [Ar] 4s0 3d2
- V(IV) - [Ar] 4s0 3d1
- V(V) - [Ar] 4s0 3d0
Chemical properties
The effect of the inner 'd' orbitals is shown in the chemical properties. Their ionization energies and atomic radii are similar, leading to similarities in reactivity. They all form double plus ions, but one of the characteristic features of the transition elements is their ability to form different oxidation states.
Their chemical properties will be dealt with in greater detail in the relevant section.
Complexes
Complexes are compounds, or ions, in which an atom (or ion) is surrounded by other species making a larger particle. The term is almost exclusively taken to mean a compound or an ion of a transition metal in which the metal ion (or atom) itself is surrounded by other atoms or ions.
This is made clearer by referring to the formulae and structures of some complexes.
![]() |
In the complex ion at the left there are six water molecules bonded to the central cobalt ion in an octahedral arrangement. The cobalt ion has a charge (oxidation state) of 3+. The water molecules are all neutral, so the overall charge on the complex ion is 3+ + 0 = 3+ The formula of the complex is written [Co(H2O)6]3+ |
The hexaamminenickel(II) ion at the right has six ammonia molecules surrounding the central nickel 2+ ion in an octahedral arrangement. The nickel ion has an oxidation state of 2+ and each of the ammonia molecules is neutral, so the overall charge on the complex ion is: 2+ + 0 = 2+ The formula is written as [Ni(NH3)6]2+ |
![]() |
![]() |
In the complex ion at the left there are four chloride ions bonded to the central copper ion in an tetrahedral arrangement. The copper ion has a charge (oxidation state) of 2+. The chloride ions all have a charge of 1-, so the overall charge on the complex ion is 2+ + 4(1-) = 2- The formula of the complex is written [CuCl4]2-. There is no need for brackets around the chloride ions. |
Bonding in complexes
The attached ions or molecules donate an electron pair to form a covalent bond to this central atom. The bonding is called dative coordinate bonding. 'Dative' because the lone pair is donated from the bonding species.
This behaviour is characteristic of the transitions metals. The first row transition elements have partially filled 'd' orbitals in the 3d level, but they also have empty 4p and 4d orbitals that can become involved in bonding. This allows the transition elements to form structures in which there are four, five or six attached species (see ligands below).
Ligands
The species that attach to the transition metal atom are called ligands (from the Latin word 'ligere' = to link). Ligands may be molecules or ions, the most common being water, hydroxide, halogens, cyanide and ammonia.
![]() |
The ligands that attach to a transition metal atom depend on the local environment of the atom. In aqueous solution the vast excess of water molecules means that the most common transition metal complex is the hexaaqua complex ion. However, ligands are 'labile' in that they can become detached and exchange places with other ligands should the conditions change. If the concentration of chloride ions is increased, for example, the complex ion may exchange the water ligands for chloride ligands. |
Some ligands form more than one attachment to the central metal ion. These are said to be chelating, or polydentate ligands. Such ligands are more effective and are found in many biological systems, for example haemoglobin.
In the haem unit of haemoglobin, there are four electron pairs donated to the central iron atom from the same 'porphyrin' group.
Note: Polydentate ligands will not be tested for first examinations 2025
Coordination number and shapes
The coordination number is the the number of attachments that all of the ligands make to the transition metal. It is the number of pairs of electrons that coordinate to the transition metal atom.
In the examples above, the hexaaqua complexes have a coordination number of 6 and the tetrachlorocuprate(II) complex has a coordination number of 4.
![]() |
The six water molecules bond to the central cobalt ion using a lone pair on the oxygen atom. There are six water molecules, so the coordination number is 6. The shape of this six-coordinated complex is octahedral. The ligands occupy all of the points of three-coordinate geometric axes. |
The copper atom has four chloride ligands bonded to the central atom. Each of the chloride ligands is bonded by means of a lone pair. The coordination number is 4. The shape of a four-coordinate complex is usually tetrahedral, although in some (rare) cases square planar complexes form. |
![]() |
![]() |
The two ammonia molecules bond to the central silver ion using a lone pair on the nitrogen atom. There are two nitrogen molecules, so the coordination number is 2. The shape of this two-coordinated complex is linear. The ligands are arranged at an angle of 180º. |
Nomenclature
The name of a transition metal complex must include the following:
- The name of the metal atom and its oxidation state.
- The names and numbers of the ligands
- The overall charge on the ion
The name of the metal atom actually depends on the nature of the charge on the ion. If the transition metal is part of a positive ion then the element keeps its usual name. eg. iron, copper, chromium etc, followed by the oxidation state.
However, if the ion is negative overall the transition metal atom changes its name (once again followed by the oxidation state):
Element | part of a negative ion |
vanadium | vanadate |
titanium | titanate |
chromium | chromate |
manganese | manganate |
iron | ferrate |
cobalt | cobaltate |
nickel | nickelate |
copper | cuprate |
The names and numbers of the ligands must be stated. The ligands have special names to differentiate them from non-complexed systems.
ligand | name |
water | aqua |
ammonia | ammine |
carbon monoxide | carbonyl |
cyanide ion | cyano |
chloride ion | chloro |
hydroxide ion | hydroxy |
oxygen molecule | oxo |
nitrogen monoxide | nitroso |
Multiplication of the ligands uses the usual di, tri, tetra, penta, hexa nomenclature.
Example: Name the following complex ion: [Fe(CN)6]3-
The overall charge on the ion is negative, so the metal name becomes ferrate.
The oxidation number needs to be worked out considering that the cyanide ion has the formula CN-. There are six cyanide ions = 6-. The overall charge on the complex ion is 3- therefore the metal ion has a charge of 3+ (3+ + 6- = 3-),i.e. oxidation state +3.
There are six cyanide ions, therefore hexacyano-
The complex ion's name is hexacyanoferrate(III)
Paramagnetism
Paramagnetism is a relatively weak magnetic force produced by unpaired electrons. It is only detectable in the presence of an external magnetic field.
The strength of the force is directly proportional to the number of unpaired electrons in an atom or ion.
Transition metal complexes do not use the 3d orbitals in bonding, leaving them able to display paramagnetism if unpaired.
The element chromium has the electronic configuration 4s1 3d5. This means that it has six unpaired electrons and a strong paramagnetic effect.
In the form of the chromium 3+ ion it has a configuration of 4s0 3d3, and consequently less of a paramagnetic effect.
In complex ions, the actual number of unpaired electrons depends on the degree of splitting of the 3d orbitals.
Colour
Colour in materials or compounds is caused when the light reaching the eyes has some of the wavelengths removed by absorption. When we see an object as red it is only this colour that is reflected from the object while the other colours or wavelengths are absorbed.
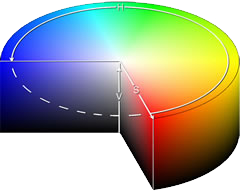
A coloured solution is caused by the white light passing through it and losing some of its wavelengths by absorption. If the solution appears blue it means that the complementary colours are absorbed by the solution.
In the complementary colour cylinder at the left, the colour that is opposite is the colour that is seen when one is absorbed.
For example, absorption of red light would leave the turquoise (cyan) colour showing. (image: wikipedia)
Coloured compounds
If colour is caused by the absorption of certain wavelengths from white light, the question remains - how are these wavelengths absorbed?
The colour in the transition metals (d-block) is usually due to the 'splitting' of the 'd' shell orbitals into slightly different energy levels. As a result, certain wavelengths of energy can be absorbed by the d-block elements (with electrons jumping between these slightly different energy levels), resulting in the complementary colour being observed.
The theory that explains the production of colour in this way is called crystal, or ligand field, theory.
This is not the only mechanism by which colour can be produced in compounds; there is also charge transfer and conjugation. Charge transfer involves the transfer of electrons from ligands to the transition metal orbitals and often produces intense colours.
One example is the deep purple colour of the manganate(VII) ion.
Conjugation is alternate double and single bonds in (usually) organic molecules, producing delocalised orbitals whose electrons can absorb light at visible wavelengths.
The azo-dyes are a good example, as are chromophores such as chlorophyll.
Crystal Field Theory
When ligands bond to transition metals they do so by donating electrons into empty hybridised orbitals.
The inner 3d orbitals are not involved in the bonding. They are, however, affected by the repulsive force of the donated electron pairs and the transition metal orientates itself to minimise the repulsions between the 3d orbital electrons and the ligands electron pairs.
When the ligands are arranged octahedrally around the transition metal ion the lowest energy orientation for the ion involves keeping the dxy, dyz and dxz orbitals in the gaps between the ligands.
However, this puts the other two orbitals, the dx2-y2 and the dz2 orbital close to the ligands, raising their energy with respect to the other three orbitals.
The 3d orbitals are now no longer degenerate. This is called crystal field, or ligand field, splitting.
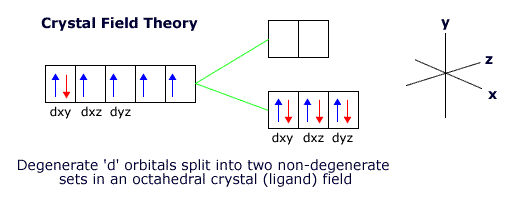
If the crystal field (the electrostatic repulsion) is strong, then the '3d' orbitals are split further with more energy between the two different sets. This depends on the ligands.
Ligands can be arranged according to the strength of their electrostatic effect into an spectrochemical series.
Factors affecting colour
Anything that can affect the electrostatic field around the transition metal ion can affect the wavelengths of light absorbed and hence the colour transmitted by a solution, or reflected by a solid.
Colour in transition metal complexes is affected by three factors:
- 1 the transition metal
- 2 the oxidation state of the transition metal
- 3 the type of ligand
The transition metals have certain colours, or colour ranges that are typical of that metal.
Copper salts, for example, are usually blue or green, iron has salts that are pale green, yellow or orange.
The oxidation state is important.
Copper(II) salts are coloured, whereas copper(I) salts are white solids.
The reason for this lies in the electronic configurations of the two oxidation states.
- copper(II) [Ar] 4s0 3d9
- copper(I) 4s0 3d10
Copper(I) ions cannot absorb energy for d-d transitions as there are no empty, or partially empty, orbitals available to accept a promoted electron.
Table of some common transition metal complex ions and their colours
Complex ion | Oxidation state of metal | colour | ligand |
---|---|---|---|
[Fe(H2O)6]3+ | +3 | pale green | water |
[Fe(H2O)6]2+ | +2 | yellow | water |
[Cu(H2O)6]2+ | +2 | blue | water |
[Cu(NH3)4]2+ | +2 | deep blue | ammonia |
[CuCl4]2- | +2 | green | chloride ion |
Note: Factors affecting the colour of a transition metal complex will not be tested for first examinations 2025
The ligand can affect the colour in two ways.
Ligands all have different crystal field strengths and will split the 'd' orbitals by differing amounts. However, apart from this, the actual shape of the complex is defined by the type of ligand and the oxidation state of the transition metal.
If the shape of the complex changes, then this also causes a change in the type of crystal field splitting. Tetrahedral fields split the 3d orbitals in a different manner to an octahedral field.
Example: Hexaaqua copper(II) ions are light blue in solution.
The complex is octahedral, and there are six water molecules coordinated to the copper ion. If concentrated hydrochloric acid is added to the solution, the water ligands get replaced by chloride ligands and a new complex, tetrachlorocuprate(II) is formed.
[Cu(H2O)6]2+(aq) + 4Cl-(aq) → [CuCl4]2-(aq) + 6H2O(l)
6-120.gif)
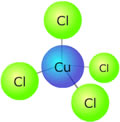
This new complex has a tetrahedral geometry and a different crystal field splitting pattern. Its colour is now a deep green as the new energy difference between the non-degenerate '3d' orbitals absorbs light of a different wavelength.
If concentrated ammonia solution is now added to the tetrachlorocuprate(II) solution, the colour fades and a light blue precipitate is formed, which then dissolves to form a deep blue solution. This final solution contains the tetrammine copper(II) complex, which is square planar.
[CuCl4]2-(aq) + 4NH3(aq) → [Cu(NH3)4]2+(aq) + 4Cl-(aq)
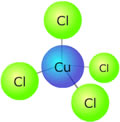
4-120.gif)